New information could become essential background reading for all of medicine.
by John Timmer
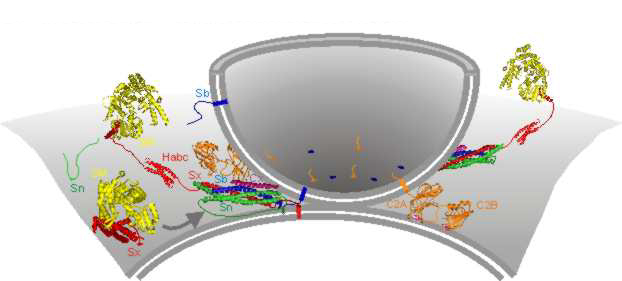
by John Timmer
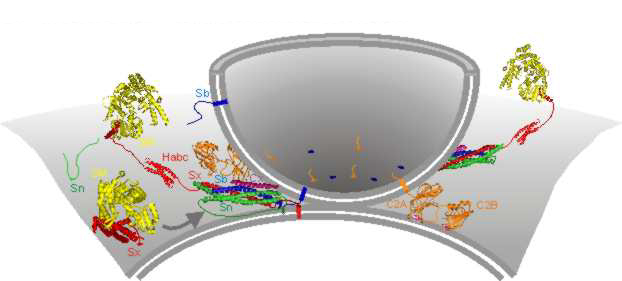
Fusing a vesicle is now known to involve a lot of proteins, shown here in color.
It's tempting to think of cells as a complex bag of water and chemicals. But cells wouldn't function if their contents were just mixed together chaotically. Instead, the inside of eukaryotic cells is carefully structured, with individual compartments performing specific functions: the DNA held in the nucleus, ATP generated in the mitochondria, damaged material digested in the lysosomes, and so on. Each of these compartments has its own specialized collection of proteins that enable it to perform these functions.
This all raises a rather significant question: how does a cell know what goes where? Or, more specifically, what happens biochemically to move proteins to the right destination, whether that destination is a specific compartment inside the cell or in the cell's environment? Three researchers have won the 2013 Nobel Prize in Physiology or Medicine for helping describe evolution's solution to that problem.
The basics of the process can be worked out with a bit of microscopy. Most of the specialized structures within a cell, as well as the cell's surface, are surrounded by membranes. Within the cell, smaller bags of membranes called "vesicles" are shuttled between structures. When they encounter their destination, the vesicles fuse with the membranes of their target, pouring their contents into it. Sorting out what goes where is mostly a matter of putting the right proteins in a vesicle and then ensuring that the vesicle fuses with the appropriate target.
In 1999, Gunter Blobel received a Nobel for figuring out the protein side of things. He showed that proteins contain short tags that help the cell determine their ultimate destination and route them using the appropriate vesicles. This year's prize goes to researchers who helped understand how vesicles move to their final destination and merge with it.
UC Berkeley's Randy Schekman turned to a simple genetic system to dissect the vesicle trafficking problem. He reasoned that destroying the transit of proteins would be fatal to the yeast, but it might be possible to find mutations that only blocked transit at elevated temperatures. His lab performed screens that first looked for high-temperature lethality and then confirmed that the lethality came about in part because proteins weren't making it to the cell's surface. Over time, he built up a collection of genes that affected this process, and by testing for interactions among them, he started dividing the process into individual pathways.
Meanwhile, James Rothman (who has spent time at too many institutions to mention; he's currently at Yale) decided to take a biochemical approach. His group isolated intact membranes from cells and found conditions in which vesicles would fuse inside a test tube. Over time, these reactions were done using ever more specific fractions of the material inside a cell. When the components were pure enough, Rothman's lab started looking at which proteins were still present.
As Rothman and Schekman started to identify the genes involved, it turned out that in many cases they were working on the same things. Vesicle trafficking was as old as eukaryotes themselves, and the same proteins were used in plants, fungi, and animals.
Combined with the work of other labs, a picture emerged where both the vesicles and their targets were decorated with a specific collection of proteins that could fit together like a lock and key (the proteins have names like SNAPs, SNAREs, and VAMPs). When the right combination of SNAPs and SNAREs is brought together, they stick to each other and cause the membranes they're embedded in to fuse. Specificity comes about because vesicles meant for one destination have a combination of proteins on their surface that can only trigger fusion with an equally specific combination on their destination.
Sometimes, however, you need more than just specificity. Cells don't send out insulin and other hormones all the time, and nerves only release neurotransmitters when they're triggered by the activity of other nerves. Stanford's Thomas Südhof shares the Nobel Prize for using the nervous system to understand how the cell controls vesicle fusion. He demonstrated that at a nerve cell's synapse, vesicles filled with neurotransmitters are poised at the cell surface, ready to fuse at a moment's notice.
Südhof identified another set of proteins that interacted with SNAPs and SNAREs, blocking their ability to trigger vesicle fusion. When the right signal was received, however—in the case of nerve cells, this involves a burst of calcium ions—the inhibition gets released, and the vesicle can fuse almost instantaneously. This process ensures that signals within the nervous system get transmitted rapidly.
The prize citation notes that this process does have some medical implications. There are a number of inherited disorders that alter the process, and some neurotoxins (including Botox) target it as well. But really, knowing something as basic as how proteins get delivered to the right place in the cell is closer to something like essential background reading for all of medicine. It helps us make sense of many, many process, a lot of which have clear and direct medical relevance.